All Features
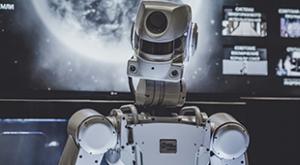
Matthew Greenwood
The next frontier for industrial digitization and automation is the convergence of artificial intelligence (AI) and machine vision.
AI-powered machine vision promises to transform the way industrial manufacturers conduct their business, according to experts at a recent webinar hosted by the…
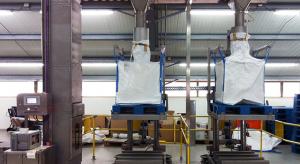
Del Williams
Manufacturers in the food and beverage sector continually seek methods to boost their operational efficiency and are prepared to invest in superior machinery when the expected returns justify the expenditure. However, the potential advantages of upgrading bulk bag filling systems are frequently…
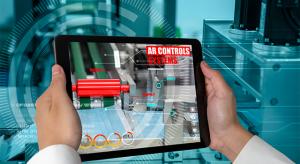
Jamie Fernandes-ETQ
Generative AI took the world by storm in 2023, from the classroom to the film studio, and the writer’s bench to the White House. Enterprises and creative industries worked to figure out how to leverage it in their operations, while classrooms and government entities struggled to govern its use.
In…
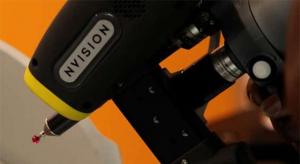
NVision Inc.
Air conditioning is hotter than ever—hot as in demand, a must-have, a comfort most of us want, and, with higher-trending temperatures generating heat-related health issues, one that many can no longer live without.
NVision, a leader in 3D noncontact optical scanning and engineering, has worked…
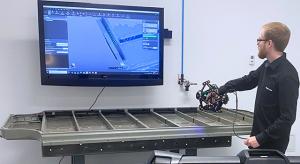
Patrice Parent
Battery trays are structural elements that enclose and safeguard the battery modules and their supporting electrical and thermal management systems. Battery modules must precisely fit into the battery tray, which must fit seamlessly within the vehicle's chassis.
Variations in tray dimensions can…
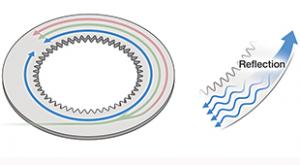
NIST
In research, sometimes the bumpy path proves to be the best one. By creating tiny, periodic bumps in a miniature racetrack for light, researchers at the National Institute of Standards and Technology (NIST) and their colleagues at the Joint Quantum Institute (JQI), a research partnership between…
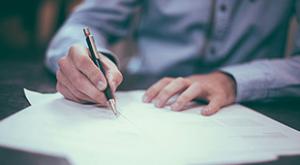
Donald J. Wheeler
Clear thinking and simplicity of analysis require concise, clear, and correct notions about probability models and how to use them. Here, we’ll examine the basic properties of the family of gamma and chi-square distributions that play major roles in the development of statistical techniques. An…
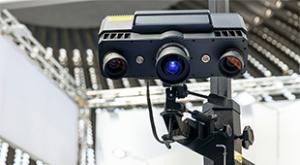
Miron Shtiglitz
The main benefit of deploying artificial intelligence (AI) for quality inspection is a significant improvement in defect detection. However, the data generated and stored by inspection systems have the potential to deliver additional benefits, including major improvements in yield.
Anyone working…
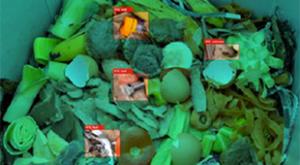
Silke von Gemmingen
Smart waste management is one of the core tasks within smart cities, i.e., those urban areas in which innovative technologies and data-driven solutions are used. They aim to improve residents’ quality of life, minimize environmental impact, and use resources more efficiently. Conserving resources…
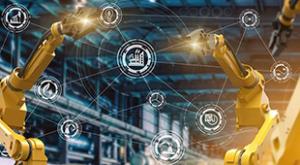
Creaform
End-to-end manufacturers are companies that lead products through the entire manufacturing process, from design to customer delivery. Unlike businesses that manufacture their products with a segmented manufacturing process, end-to-end manufacturers have complete control over the different parts of…
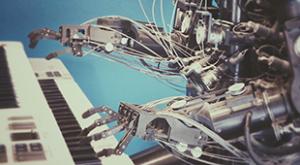
Eric Whitley
High-precision manufacturing is critical in industries where even the slightest deviation can lead to significant consequences. It encompasses processes that demand the utmost accuracy, often in sectors like aerospace, medical devices, and electronics. Precision is important due to its direct…
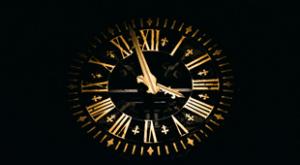
Andrew Novick
While people around the country are preparing champagne and getting ready to watch the ball drop on New Year’s Eve, I’m closely monitoring our clocks at the National Institute of Standards and Technology (NIST).
We actually monitor them every nanosecond of every day, not just on New Year’s Eve.…
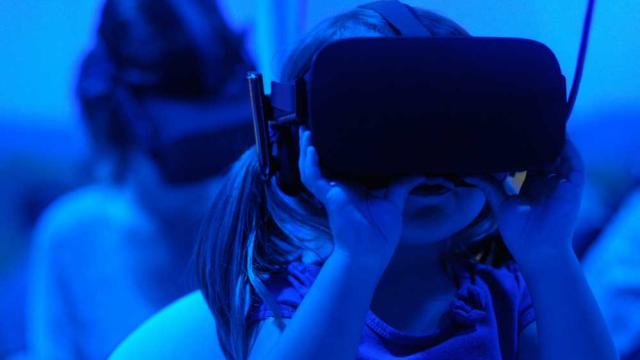
Jason Walker
As the manufacturing industry continues to grapple with labor shortages, potential shifts in government policies could further intensify these challenges in 2025. This heightens the urgency for manufacturers to meet demand while navigating an unpredictable future.
One area where this is…
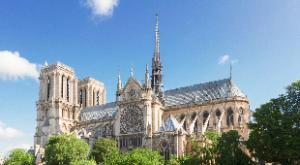
Industrial Inspection and Analysis
Unlike the traditional engineering process of designing a part, product, or component from the ground up, many times in life we need to start with an existing item and work backward to solve a problem. It’s a process known as reverse engineering, and it begins by obtaining accurate data about the…
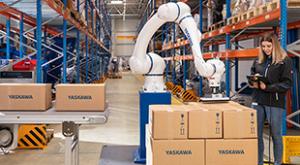
Chris Caldwell
The quest to optimize equipment efficiency, ensure consistent quality, and lower operational costs while empowering a stronger workforce continues to permeate the industrial landscape. While challenges persist, the transformative potential that high-performance robotic automation is bringing to…
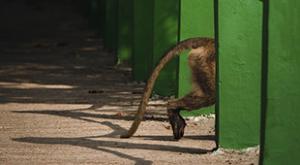
Donald J. Wheeler
When do we need to fit a lognormal distribution to our skewed histograms? This article considers the basic properties of the lognormal family of distributions and reveals some interesting and time-saving characteristics that are useful when analyzing data.
The lognormal family of distributions…
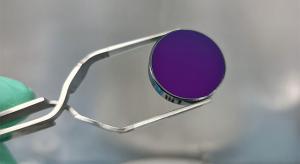
University of Vienna
In the field of high-performance mirrors, everyone chases the impossible: coatings with perfect reflectivity. In the visible range of wavelengths (i.e., between 380 nm and 700 nm), advanced metallic mirrors achieve reflectivities as high as 99%, which means 1 photon is lost for every 99 reflected.…
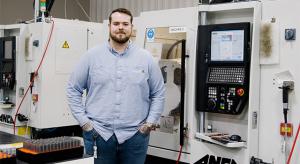
Russell Riddiford
Whether you use a lot of cutting tools or sometimes find yourself desperately lacking the right tool, you’ve probably considered making your own—or at least sharpening your worn tools. But how do you decide if taking tool grinding in-house is the smart decision? It’s a question GKN Aerospace Engine…
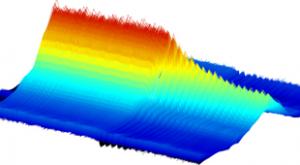
NIST
From monitoring concentrations of greenhouse gases to detecting Covid in the breath, laser systems known as frequency combs can identify specific molecules as simple as carbon dioxide and as complex as monoclonal antibodies with unprecedented accuracy and sensitivity. Amazing as they are, however,…
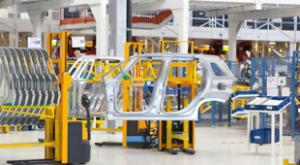
Creaform
As manufacturers transition toward Industry 4.0 to speed up production cycles and accelerate their time to market, they nevertheless continue to face many challenges, particularly with respect to automating quality control.
Reducing costs drives the need for automated quality control
Automating…
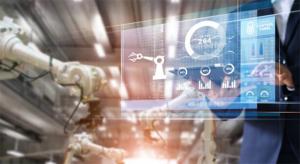
Nicolas Lachaud-Bandres
Imagine a factory where quality assurance actually increases the production speed. Advanced metrology equipment is well on the way to making this a reality by introducing new levels of connectivity with other pieces of equipment and software throughout the factory.
Communication between different…
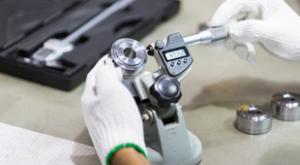
Master Gage and Tool Co.
Calibration is essential in almost every facet of industrial processes. The calibration process verifies test instrument accuracy by comparison with recognized standards, and measurement validity hinges on one crucial concept: traceability.
Traceability adherence ensures a continuous link between…
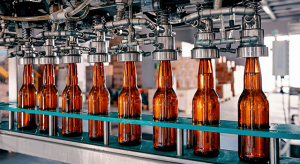
Scott A. Hindle, Douglas C. Fair
Parts 1, 2, and 3 of our series on statistical process control (SPC) have shown how data can be thoughtfully used to enable learning and improvement—and consequently, better product quality and lower production costs. Another area of SPC to tap into is that of measurement methods. How do we ensure…
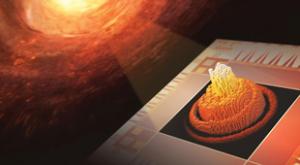
NIST
Researchers at the National Institute of Standards and Technology (NIST) and their colleagues have built a superconducting camera containing 400,000 pixels—400 times more than any other device of its type.
Superconducting cameras allow scientists to capture very weak light signals, whether from…
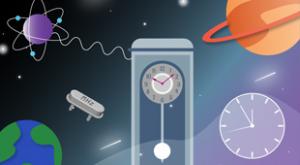
Tara Fortier
Measuring and keeping track of time has allowed people to plan and coordinate locally with others for millennia.
As the world has modernized and our pace of life has increased, our relationship to time has changed. Time has become a scarce commodity. The standardization of time has also become…