All Features
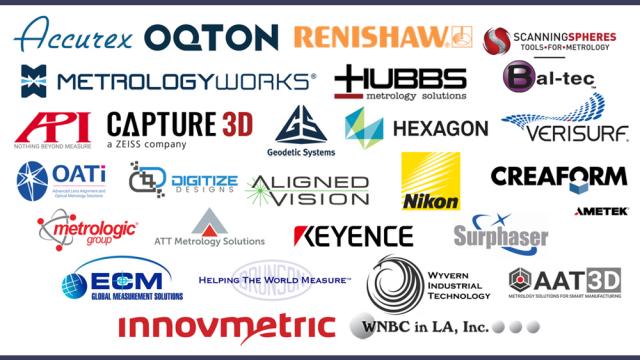
Megan Wallin-Kerth
As the July 22–25, 2024, Coordinate Metrology Society Conference (CMSC) rapidly approaches, quality professionals anticipate opportunities to explore and showcase various products and ideas from some of the biggest names and organizations. InnovMetric, Hexagon, Renishaw, Nikon, and many others will…
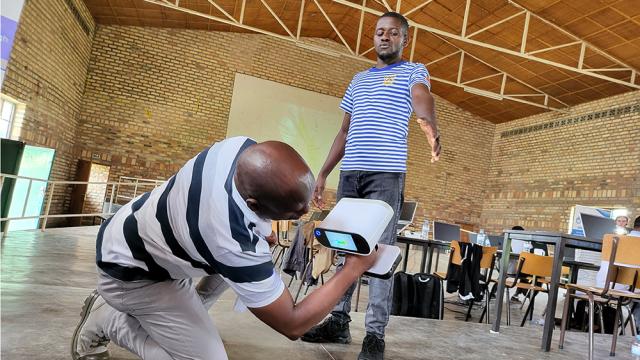
Artyom Yukhin
Artec 3D has begun working with Handicap International to manufacture custom prosthetic devices for beneficiaries in refugee camps and their host communities in Rwanda.
According to the United Nations, in Rwanda more than 51,000 people need prostheses. Many suffered loss of limbs during the 1994…
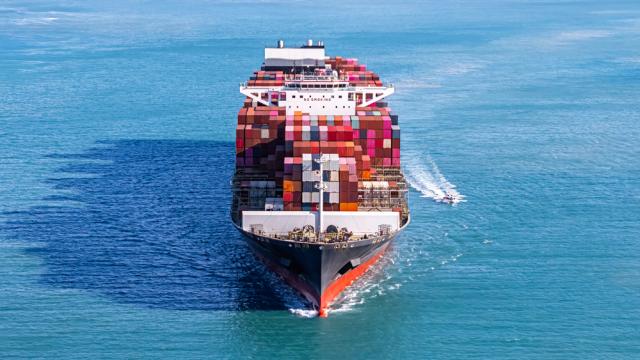
Georgia Tech News Center
When people think of greenhouse gas emissions from transportation, what often comes to mind are airplanes and land vehicles like cars or trucks. But as efforts to slow climate change are ramping up, the spotlight is on another form of transport: ships.
The United Nations’ International Maritime…
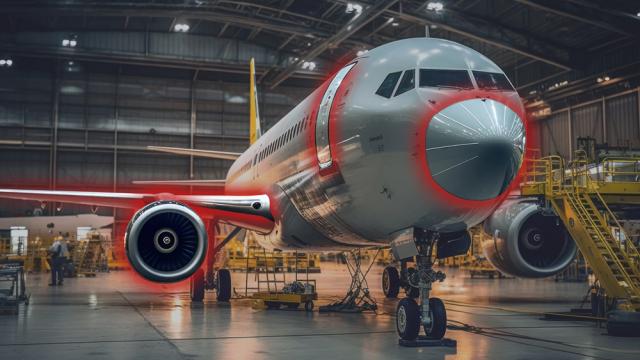
Creaform
When it comes to aircraft, poorly documented dents can lead to more significant problems, potentially compromising structural integrity or performance. Dents can trap moisture and lead to corrosion. The stress they generate can initiate fatigue cracks. Their effects on the structure can also affect…
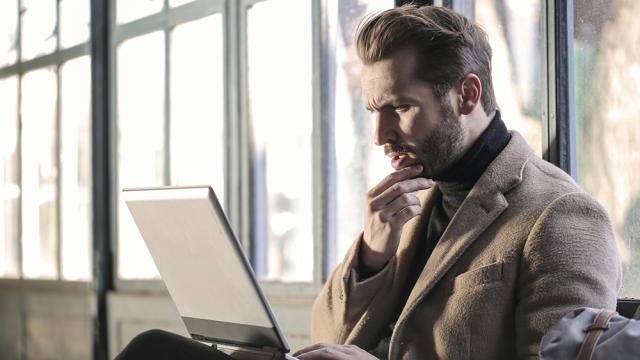
Donald J. Wheeler
The four common capability and performance indexes collectively contain all of the summary information about process predictability, process conformity, and process aim that can be expressed numerically. As a result, any additional capability measures that your software may provide can only…
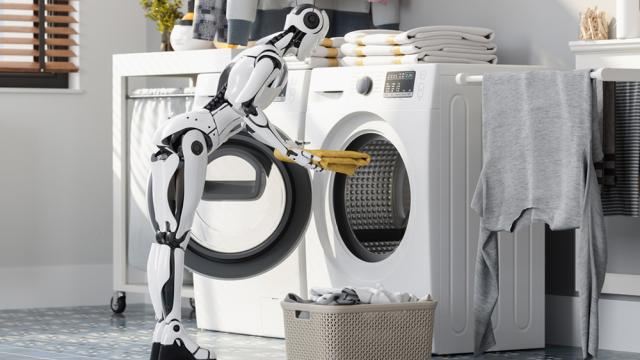
Adam Zewe
Someday, you may want your home robot to carry a load of dirty clothes downstairs and deposit them in the washing machine in the far-left corner of the basement. The robot will need to combine your instructions with its visual observations to determine what it should do to complete this task.
For…
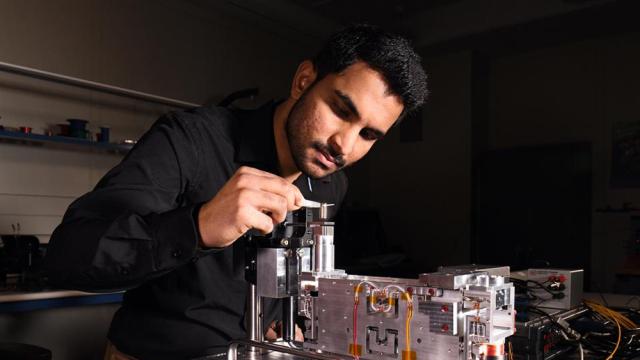
NIST
Bringing its cutting-edge invention to the larger world for the first time, the National Institute of Standards and Technology (NIST) has delivered a portable and super accurate tabletop instrument for measuring mass to the U.S. Army. This could revolutionize critical measurements in a variety of…
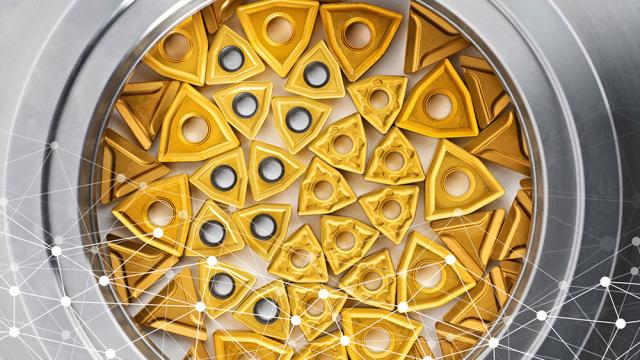
Silke von Gemmingen
Indexable inserts are interchangeable cutting tools that are indispensable in various industrial applications, especially in metalworking. They are used as cutting material carriers for machining metals, plastics, or wood.
Manufacturing indexable inserts requires high-precision production…
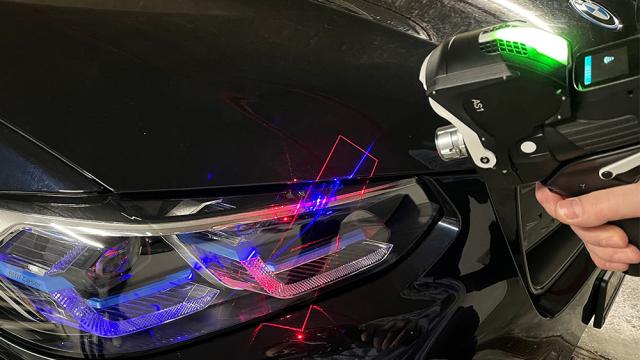
Pirmin Bitzi
Data are at the core of smart manufacturing; they’re pivotal to innovation and agility. At the same time, the vast amounts of new data captured daily in manufacturing present substantial challenges—no more so than within quality control, where this significant data load can lead to data analysis…
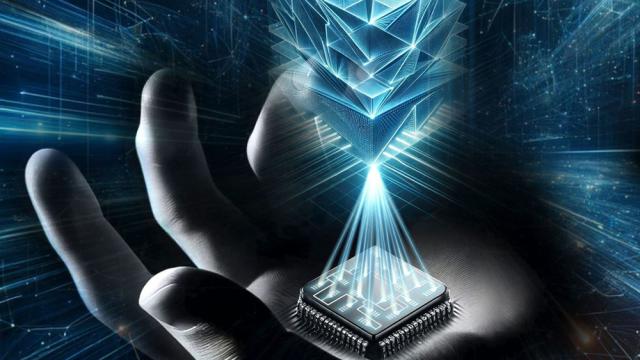
Adam Zewe
Imagine a portable 3D printer you could hold in the palm of your hand. This tiny device could enable you to rapidly create customized, low-cost objects on the go, like a fastener to repair a wobbly bicycle wheel or a component for a critical medical operation.
Researchers from MIT and the…
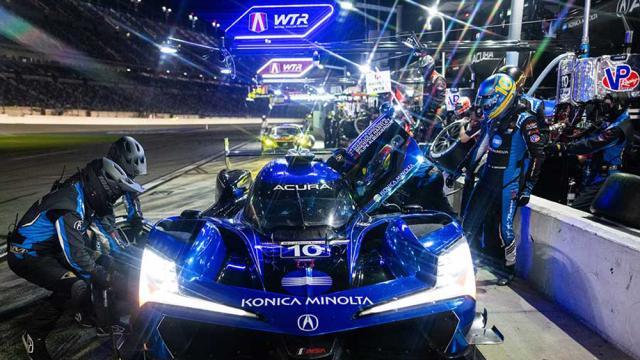
AMETEK
Endurance racing is one of the oldest and toughest pastimes in motorsports. It is a true test of performance. Not only is a driver’s stamina on display, but vehicle durability as well.
Based in Indianapolis, Wayne Taylor Racing With Andretti (WTRAndretti) is a world-renowned global motorsports…
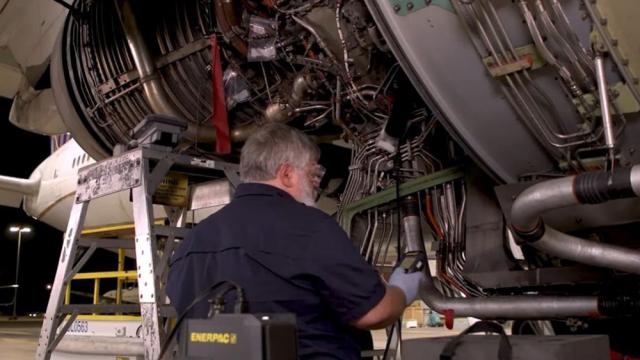
Masateru Ito
For organizations responsible for aircraft operation and maintenance, inspecting critical aircraft-engine components is a routine preventive maintenance procedure. These procedures help to ensure the proper operation of the aircraft for flight safety. There are many components inside the engine…
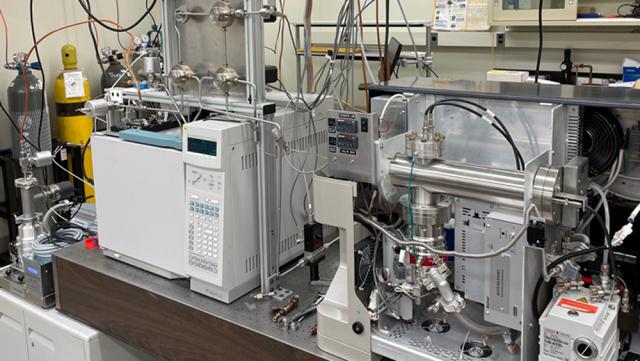
NIST
To combat global warming, companies are building direct air capture (DAC) facilities worldwide to remove carbon from the atmosphere. The National Institute of Standards and Technology (NIST) has developed a new method for testing the materials used in these plants to capture carbon. The agency…
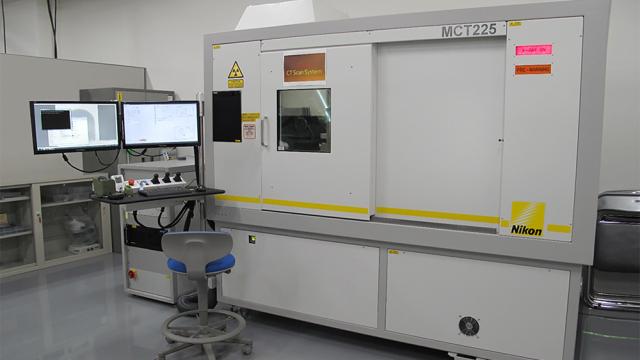
Nikon Metrology Inc.
Nikon’s X-ray computed tomography (CT) technology has enabled digital camera lens heavyweight Tamron to gain unprecedented insights into the intricacies of its industry-leading components.
Tamron faced challenges in accurately analyzing the deformation of plastic molded lens components. Nikon’s…
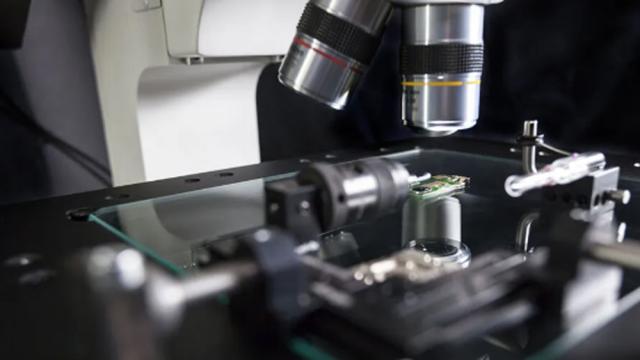
Mitutoyo Corp.
Choosing the right microscope for your application is no small feat. It’s a decision that requires a deep understanding of the task at hand and the tools available to you. From magnification to resolution, and from ease of use to customization options, there are several aspects to consider when…
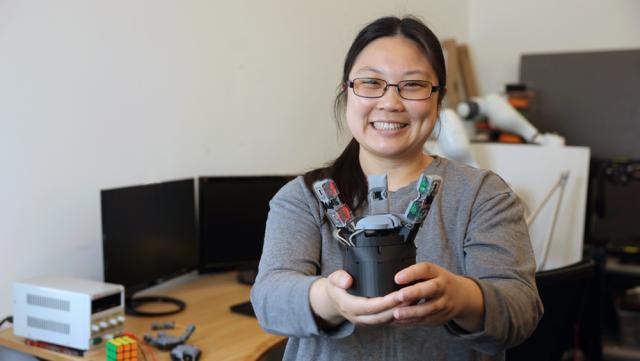
Rachel Gordon
‘I’ll have you eating out of the palm of my hand” is an unlikely utterance you’ll hear from a robot. Why? Most of them don’t have palms.
If you have kept up with the protean field, gripping and grasping more like humans has been an ongoing, Herculean effort. Now, a new robotic hand design…
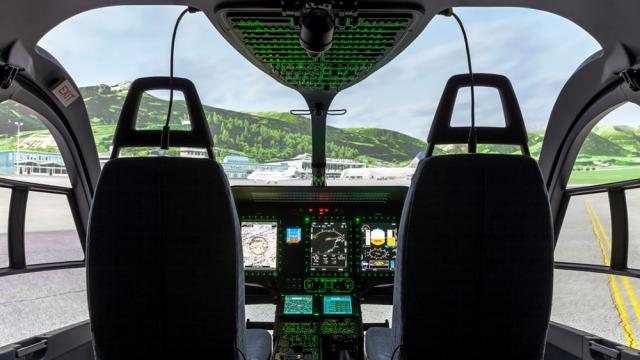
Q.Big 3D
Additive manufacturing of oversized plastic components offers enormous advantages for mold-free small- and medium-size production runs. The key to this is Q.BIG 3D’s VFGF (variable fused granulate fabrication) process.
Reiser Simulation and Training, in Berg near Starnberg, Germany, commissioned…
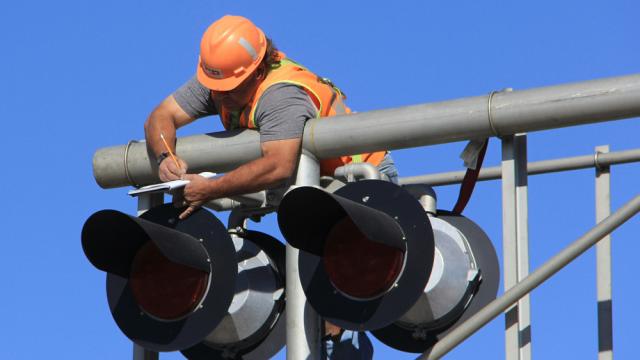
James Chan
Preventive maintenance (PM) is a proactive maintenance strategy built on calendar-based maintenance tasks, regular inspection, and preemptive repair of physical assets. Physical assets may refer to equipment, production machinery, and operational facilities. Preventive maintenance tasks are…
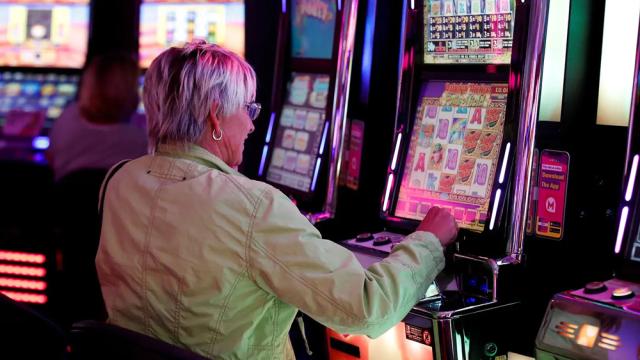
Katie Gilbert
Researchers at Stanford Graduate School of Business have pushed past the limitations of A/B testing into another area of experimentation focused on “multi-armed bandits.” Mohsen Bayati, a professor of operations, information, and technology who has been exploring these problems for the past 15…
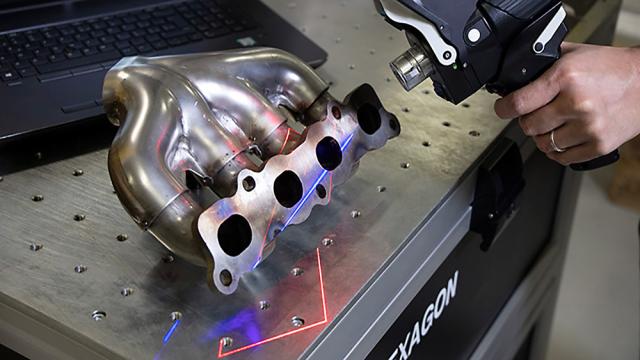
Anthony Vianna
Over the past decade, technological improvements have seen 3D laser scanning become an increasingly important alternative to tactile measurement in an expanding range of measurement applications.
The success of laser scanning owes as much to under-the-hood technologies that improve the usability…
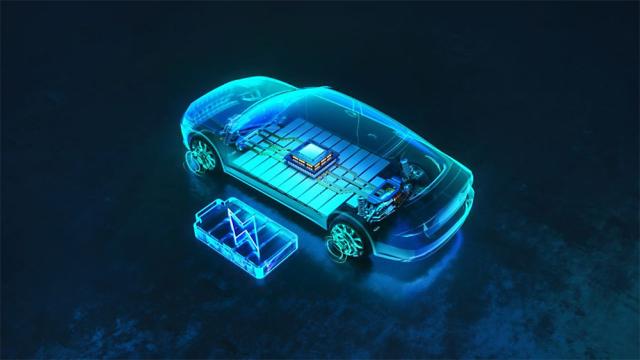
Seunghak Lee
Global electric vehicle (EV) sales have been growing steadily and are expected to reach more than 17 million vehicles in 2028. The rapid shift toward EVs means that new inspection solutions are needed to ensure the quality of critical components. For electric cars, these components include the…
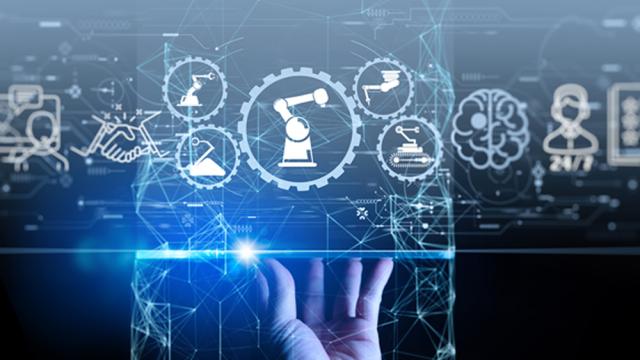
Michael Sharp
American manufacturing is associated with high-quality standards that are meant to ensure both the reliability and longevity of the products produced. Manufacturers across all industries are looking for technological solutions and enhancements to continue to meet these high-bar standards and to…
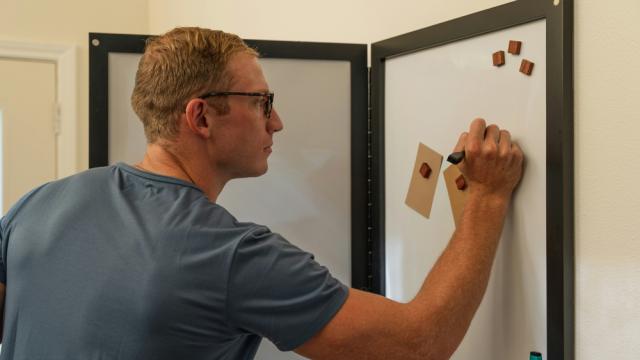
Henry A. Zumbrun
We’ve been asked by customers how many decimal places are enough. It’s either because they want to maximize the resolution of their purchase or they expect far more resolution from the equipment than is reasonable.
We’ve seen a lot, even a meter being set to read 100,000.001. That’s 100 million…
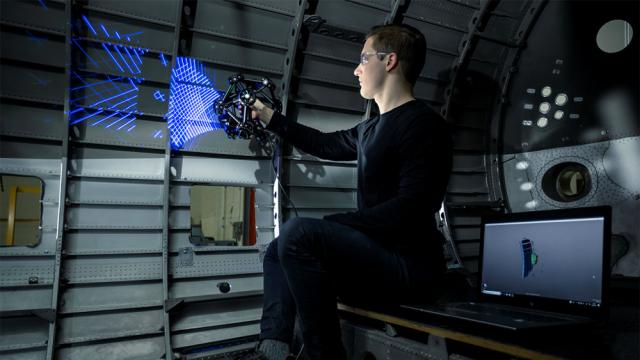
Simon Côté
Integrating 3D scanning technology into quality control processes can be challenging. It requires balancing the need for cost-effective, scalable solutions with long-term effectiveness.
We’ve compiled a list of our best tips to help you choose a 3D measurement technology that meets your current…
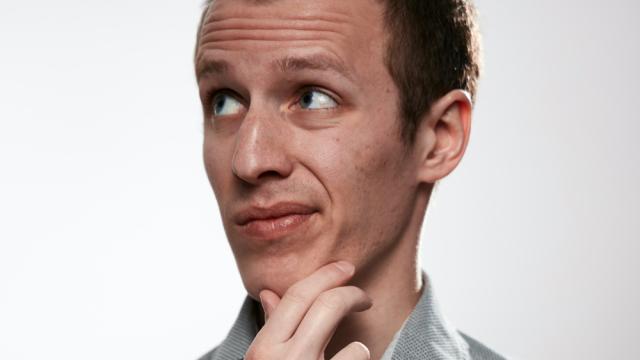
Harish Jose
In this article, I’m looking at the relationship between capability index (Cpk or Ppk) and tolerance intervals. The capability index is tied to the specification limits, and tying this to the tolerance interval enables us to use the confidence/reliability statement allowed by the tolerance interval…