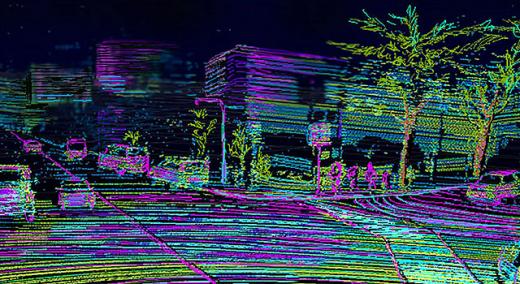
Confusion between the two terms “RADAR” and “LIDAR” is understandable. Their names are nearly synonymous, and the terms are often used interchangeably. The acronyms are RADAR, which stands for RAdio Detection And Ranging; and LIDAR, which stands for LIght Detection And Ranging. The major difference between the two is the wavelength of the signal and the divergence of the signal beam.
ADVERTISEMENT |
LIDAR is typically a collimated light beam with minimal divergence over long distances from the transmitter; RADAR is a cone-shaped signal fanning out from the source. Both calculate distance by comparing the time it takes for the outgoing wave or pulse to return to the source. LIDAR uses light wave frequencies that have a shorter wavelength, which enhances the capability of collecting data with high precision. RADAR uses longer microwave frequencies, which have lower resolution but the ability to collect signals with reduced impact from environmental obstructions. RADAR and LIDAR signals both travel at the speed of light.
…
Add new comment