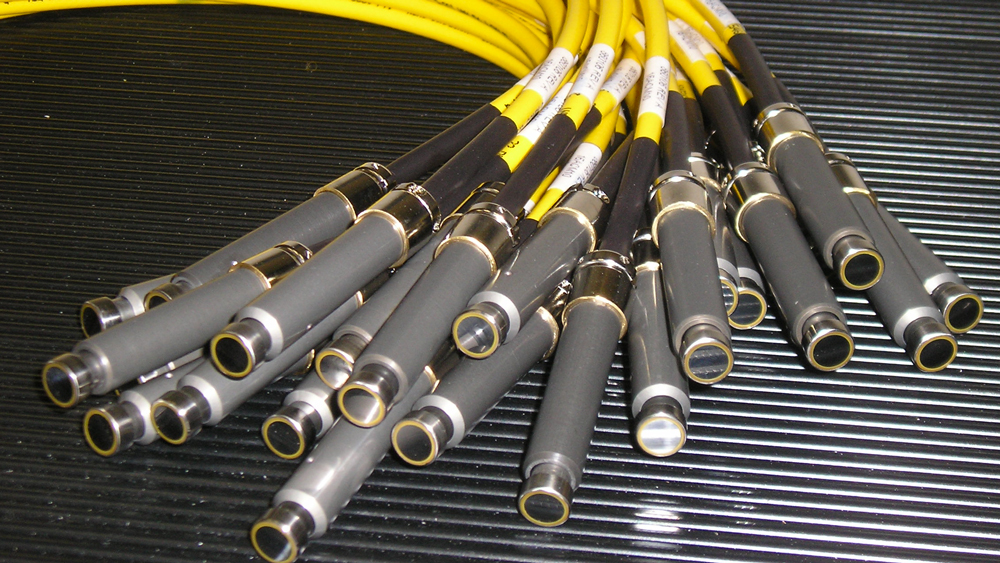
In-process temperature sensors
In 1988, a small company began developing and supplying electronic instruments that automatically compensate for temperature-induced errors in industrial gages that are used to make precision dimensional measurements. Its products are now in use worldwide, improving factories and workshops that machine metal parts to tight tolerances.
ADVERTISEMENT |
ISO 1, the first standard issued by the International Organization for Standardization (ISO), specifies the standard reference temperature for geometrical product specification and verification, which is fixed at 20°C (68°F). This temperature can be difficult to maintain in a manufacturing environment, and all the elements of a measuring system (workpiece, master, and gage fixture) can be affected by thermal influences.
When certain parts are measured after precision machining or cleaning operations, or after otherwise being exposed to temperatures other than the reference temperature (20°C/68°F), their dimensions can be significantly altered by thermal expansion or contraction. Gage fixtures and masters might also not be at reference temperature. The result will be that erroneous measurements will be made unless this is taken into consideration.
…
Add new comment